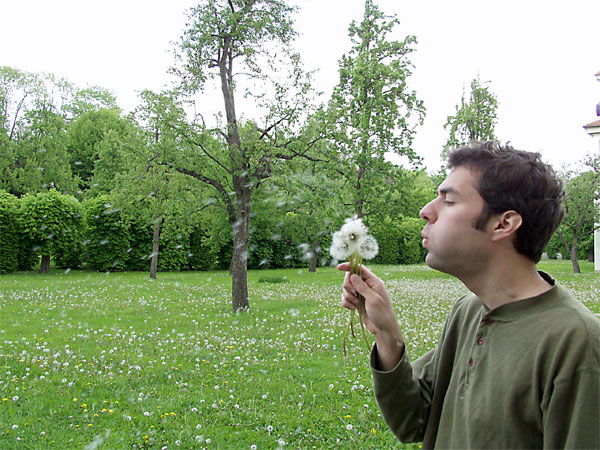
PLANT INVADERS AND TERRESTRIAL PHYSIOGNOMY
by Anthony Darrouzet-Nardi · 14 December 2004
Introduction
he word physiognomy is a synonym for countenance or facial expression and is used in a similar sense by botanists to mean the general character of the plant landscape. Terms like sagebrush steppe, maritime chaparral, mixed conifer forest, and early-successional old field describe landscape physiognomy. There is no equivalent for the word physiognomy in zoology, perhaps because a landscape--given that it is sufficiently unaltered by human activity--is defined by its vegetation: plants are what you see in the landscape. During our rapid rise to global ecological dominance, humans have appropriated half the terrestrial surface for agriculture, forestry, pasture, and settlement (FAO 2001). We have altered the Earth's cycles of nitrogen, water, and carbon dioxide (Schlesinger 1997). A combination of these factors is changing Earth's climate (Houghton 2001). Finally, we have dispersed species far beyond their unaided potential, allowing spectacular biological invasions (Vitousek et al. 1996, Mack and Lonsdale 2001).
Invasion ecology has recently become a favored research topic for ecologists, perhaps because existing theory from studies of successional dynamics and disturbance processes can be readily applied. Several years ago at the Ecological Society of America annual meeting, I estimated that about one third of presentations were related to invasions. There has also been a spate of high-profile review papers emphasizing the importance of plant invaders to Earth's ecosystems and human society (e.g., Mack et al. 2000, Pimentel et al. 2000). An observant historian or other non-ecologist might ask if non-native invasive species are really a formidable threat to the Earth, or if this is the latest chapter in the declensionist narrative (see Cronon 1992). In response to this criticism, many ecologists have thus refocused on the more scientifically appropriate question, "How are humans changing the nature of the Earth?" With this question in mind, this essay aims to review the significance of plant invaders to terrestrial physiognomy.
Definitions
The literature of invasion ecology is replete with adjectives to describe the organisms in question: invasive, exotic, non-native, introduced, alien, weed, etc. (Pysek 1995). In considering the effects of plant invasions on a global scale, there are two important phenomena to consider: First, humans are dispersing plants beyond their unaided potential across oceans, mountains, deserts, and other barriers. I will call these human-dispersed plants "non-native." Second, humans are modifying the environment so as to encourage invasions. Although the word "invasion" connotes hostility (of which plants are not capable), I will use it as a demographic term to describe a rapid expansion in abundance of an organism or group of organisms that culminates in dominance of a landscape. Note that an invasion could occur by either a native or a non-native organism. An important wrinkle arising from these two definitions is that non-native plants as a group might be said to be invading Earth's ecosystems due to their rapid rise in abundance. Finally, words like "weed" and "pest" refer to things that humans do not like. One man's weed is another's prized ornament, making this concept difficult scientifically. (Though, it is interesting to note that most recognized agricultural weeds (see Holm 1997) are non-native (Mack and Lonsdale 2001).)
1. Wind, water, and wing. Don't plants disperse naturally?
Traditionally, the first rebuttal to an assertion of substantial human impact on the Earth is that the phenomenon in question (e.g., global warming, biodiversity loss, etc.) is a natural phenomenon that occurred well before humans. This argument is then countered by the fact that while the phenomena are natural, humans have pushed their rates outside the historical range of variability. Global dispersal of plants fits this pattern well. Mack and Lonsdale (2001) review the literature comparing natural dispersal rates with human-aided dispersal. They conclude "Natural forces thus represent a vanishingly small threat as vectors for the transoceanic movement of weedy species."
Mack and Lonsdale (2001) also separate human-aided plant dispersal events into three categories: accidental, utilitarian, and aesthetic. Utilitarian introductions of non-natives primarily include plants that are used for food, fiber, and animal forage. Aesthetic non-natives include ornamental plants for gardens or plants brought to introduce a botanical flavor from a previous home. We most often imagine a seed inside the mud stuck on a boot as the type of event that has accelerated the global dispersal of plants; however, deliberate introduction of crop plants and ornamentals for gardens are historically more important and they continue to be the dominant mode of global plant dispersal.
In 1986, historian Alfred Crosby wrote a book entitled Ecological Imperialism: The Biological Expansion of Europe, 900-1900 (Crosby 1986). This book discusses the role of the European biota that was transported by colonists during colonial era. Crosby's work emphasizes that not all dispersal routes are equal. Some areas of the globe, especially those that were colonized by Europeans were more actively impacted by utilitarian and aesthetic introductions.
2. How much of the Earth do non-natives cover?
Human alteration of terrestrial Earth spans a spectrum of subtlety. Laying pavement for roads and tilling fields for agriculture change Earth's physiognomy such that at a glance we can see our impact. Forestry and ranching are subtler--a closer look can be necessary to see if an ecosystem has been logged or grazed. Boreal forests and alpine tundra experience effects that are subtler still, yet it is conventional environmental wisdom that no ecosystem is pristine (Cronon 1995). The effects of human-dispersed plants span the range from subtle to severe. Sometimes plant invasions are on the order of the subtle changes caused by elevated [CO2] or low-level nitrogen deposition. For example, Taraxacum officinale (dandelion) is ubiquitous in North America, but it does not dominate landscapes. It is the only appreciable non-native plant in the flora of the subalpine meadows of the Sierra Nevada where it comprises <1% of the plant cover (Odion et al. 1988). Eucalyptus species transplanted from Australia are a stronger visual force on the landscape of the central coast of California, but they do not spread themselves rapidly and could probably be removed if so desired. On the other hand, non-natives such as Pueraria montana (kudzu), Bromus tectorum (cheatgrass) and Centaurea spp. (star thistle, diffuse and spotted knapweeds) have become "superinvaders" and are altering landscapes on the order of the changes caused by pavement and agriculture.
The most basic datum for determining the effect of plant invaders on terrestrial physiognomy is the percent of the global plant canopy cover occupied by non-native species. Knowing this, we could then go on to determine how different the landscape looks with these non-natives. Are native species typically displaced by similar-looking and closely related non-natives, as when two annual grasses are switched? Or are functional groups switched as when annual grasses replace perennial grasses? Or are ecosystem types drastically changed as when trees invade a grassland, turning it into a forest? Unfortunately, this datum is unavailable. As Richard Mack explains, "Plant invasions well illustrate our dilemma in assessing the profound global changes that biotic invasions in general hold for environments and economies: we usually lack comprehensive and accurate records that even characterize these species' aerial extent, much less document the population dynamism throughout the history of their new range" (Mack 2000). Remotely sensed data is not of a high enough resolution to distinguish individual plant species and non-native species have no distinctive signature that we can measure. Assessing species composition on the ground is too time-intensive to apply to the entire terrestrial surface.
Despite these limitations, there are several ways that we can gauge the impact of non-native plants on terrestrial physiognomy. The first is using proxy measurements for non-native success. Jenkins and Pimm (2003) argue that of the variables for which we have data, human disturbance to the landscape is the best correlate with weed success (n.b. they discuss "weeds" instead of non-natives, but recall that most weeds are non-native). They use remotely sensed data to determine which areas on Earth are most substantially affected by human activities and label this portion of the Earth the "global weed patch." (Their figure is shown below.) The global weed patch includes "croplands, mosaics of croplands and natural vegetation, urban areas, and original tropical woodlands and forests that are now grassland savanna or woody savanna" Since there is no explicit plant data, this type of analysis gives only a hint at the global percent plant cover of non-native species. However, it does suggest where the percent cover will be higher and lower. I suspect that there is substantial difference in non-native cover between the disturbed and undisturbed areas of their projection. Some areas, such as croplands, are surely covered with non-natives (the crops themselves). However, for the half of the globe that has not been directly appropriated for human use, this method does not seem to work as well. For example, the Great Basin area of the Western United States is known to have a high percent cover of cheatgrass, knapweed and other non-natives, yet it shows up as outside the weed patch.
The second method for gauging the impact of non-native plants on terrestrial physiognomy is a sampling approach. Lonsdale (1999) assembled data from a wide variety of vegetation studies that listed both the native and the non-native flora and estimated a global percent of species that are non-native. The mean global fraction of non-native species in Lonsdale's sample was 16%, ranging from 1.3% in the Zachariashoek reserve in South Africa to 64% in Hawaii Volcanoes National Park. If we assume that species richness correlates well with aerial cover and we assume that Lonsdale's sample is a random selection from the globe, then 16% would be the global percent cover of non-native species. However, richness may not correlate with aerial cover and ecologists do not choose their field sites or people their nature reserves by throwing darts at a spinning globe. Lonsdale does note that his data set has "imperfections (e.g., poor coverage of South America and Asia)," but it is informative nonetheless. From it, we can say that non-native plants are 1) just about everywhere and 2) the dominant component of the flora in some areas.
3. How will other agents of global change affect plant invasions?
As described in the introduction, other agents of global change include land-use change, habitat fragmentation, rising atmospheric [CO2], increased rates of nitrogen cycling including increased rates of atmospheric deposition of ammonium and nitrate, and climate change. Dukes (1999), in a summary of the effects of these changes on invasive species, concludes that all of them will tend to increase the rate of species invasion, with the possible exception of increasing atmospheric [CO2], which he contends will favor some invasions and disfavor others. Ecological theory suggests that when the resource environment or disturbance regime of an ecosystem is substantially altered, species composition change should follow. The question of whether non-native invaders per se will be favored is a more difficult question since we have not discovered any clear defining characteristics that separate natives from non-natives. I will discuss several examples that demonstrate how various global change agents seem to have encouraged non-native plant invasions.
Earlier this year, I worked at the Nevada Desert FACE Facility in the Mojave Desert (FACE stands for free-air CO2 enrichment). This facility is part of a network of experiments examining the effects of elevated [CO2] in the field. They have three 23 m diameter rings in which they have elevated [CO2] to 550 ppm and three rings left at ambient [CO2] (375 ppm). When it does not rain in the Mojave Desert, which is usually the case, very little of ecological interest occurs. The biota wait for rain and try to conserve resources. When it does rain, plants and soil crusts begin to fix carbon and cycle nutrients rapidly. Additionally, the seed bank, replete with annual plants, will recruit. During the 1997-1998 El Ni–o event, when the Mojave Desert received 2.4 times the average annual rainfall, the annual plants sprouted and set seed. Researchers at the FACE site quantified the density, biomass, and seed rain of four annual plants: three native plants, and the non-native invasive Bromus madritensis (red brome) (Smith et al. 2000). They found that in the rings in which [CO2] was elevated, B. madritensis was much more successful than in the rings with ambient [CO2] while the three native annuals did not respond to the treatment as greatly.
The global nitrogen cycle is arguably the most human-altered of the major biogeochemical cycles. Fertilizer production, planting of N-fixing crops, and fossil fuel combustion have more than doubled global fluxes of N (Vitousek et al. 1997). On land that does not receive the direct inputs of fertilizers or N-fixing crops, the most important change to the nitrogen cycle is the increase in atmospheric N deposition. Ecosystems vary in the amount of N deposition they receive, with heavily industrialized regions receiving the highest rates of deposition (Galloway et al. 1995). The main sources of atmospheric N are fossil fuel combustion and volatilization of agricultural byproducts. Atmospheric N can travel hundreds of kilometers and land in otherwise isolated ecosystems. Since N is one of the most limiting resources for plant growth, especially in temperate ecosystems (Vitousek and Howarth 1991), N deposition might be expected to affect plant invasion dynamics particularly in temperate systems. For example, a study in the acid grasslands of England (Stevens et al. 2004) has shown a remarkable correlation between N deposition rate and the species richness (4 m2 scale) of the grassland. They measured a transect of 68 grasslands and found that when regressing N deposition against plant species richness, they accounted for 55% of the variance in the data. The coefficient was such that for every approximately 2.5 kg N ha-1 yr-1 that was deposited, there was one less species, thus implying some amount of invasion by the better competitors. Looking more specifically at non-native invaders, a study of N requirements in plants from the German flora shows that the non-natives tend to have higher N requirements than natives, suggesting that N deposition is abetting the non-native invasion of Germany (for once) (Scherer-Lorenzen et al. 2000).
In the above two biogeochemical examples, global anthropogenic impacts to element cycling appeared to be encouraging invader success, but it is harder to imagine how invaders would feed back to global [CO2] or rates of N deposition. However, in the case of altered disturbance regimes, it is well documented how non-native invaders, especially grasses, can greatly modify the fire cycle (D'Antonio 2000). Although non-native grass invasions may be able to create a positive feedback between grass success and fire, there is usually a human disturbance such as land clearing involved in the creation of the new fire regime (D'Antonio and Vitousek 1992). New fire regimes can reduce the fire return interval by as much as an order of magnitude. Changes to landscape physiognomy in this type of scenario are dramatic.
One question that these examples of non-native invasions in response to global change agents bring up is: why are the non-natives successful instead of strong local competitors? There are many possible reasons that certain non-natives may be able to perform better than natives given these global change factors including the introduction of novel allelopathic weapons (Callaway and Ridenour 2004), release from native consumers, and superior pre-adaptation for resource preemption in the invaded environment among others (Mack 2000). However, we should also remember that most non-natives are not successful in new environments; we usually only notice the ones that are successful.
Finally, when considering the effects of these global change factors on plant invader success, and consequently on terrestrial physiognomy, it is important to remember that these changes are acting not alone, but in concert. Their effects will not be directly additive (e.g., Zavaleta et al. 2003). The one major change that I did not discuss is climate change, which is perhaps the hardest to predict. Investigating the effects of the expected global warming of several degrees centigrade over the next century is not intractable, but this effect will probably pale in comparison to changes in precipitation regimes. Water, like nitrogen, frequently limits plant growth and thus its modification can be expected to have large impacts on terrestrial physiognomy. Unlike temperature, which will likely go up nearly everywhere on the globe, changes in precipitation will be spatially variable, with some areas becoming wetter and others drier. And none of this takes into account the truly magnificent changes that might accompany an abrupt climate change event (NRC 2002). Trying to factor in such an important effect in concert with all of the other effects makes predictions impossible at present. In reality, we could likely discover examples where global change factors are actually reducing invaders, but taken together, if anything, human impacts to the globe are going to increase plant invasions. At the very least they won't affect them much.
4. What are the effects of plant invasions on ecosystems?
All plants have the same basic physiology: they fix carbon from the atmosphere, grow, and reproduce themselves. Nutrients such as nitrogen and phosphorus are used by plants to construct macromolecules, which include the protein machinery that performs most biological functions. Furthermore, the protein machinery that runs photosynthesis, where plants invest most of their protein, works approximately the same in all land plants (Lambers et al. 1998). Thus, we might expect all plants to process nutrients in a similar manner. However, the rate at which plants use their machinery--their metabolic rate--can vary tremendously between organisms. Steltzer (1998) showed that rates of N mineralization and nitrification under two co-dominant alpine species on Niwot Ridge (Acomastylis rossii and Deschampsia caespitosa) differed by as much as several orders of magnitude (see her Figure 1). These two species were interspersed and not in any apparent successional progression (some of the plants are hundreds of years old). This type of difference can have substantial effects on local nutrient cycling, especially since litter, rhizosphere dynamics, and the underlying microbial community can all contribute to a conservative or non-conservative N cycling regime (Hobbie 1992). Thus, when new plant species invade an area, we can expect that in some cases ecosystem function will changed substantially.
While plant invasions are frequently abetted by human alteration of the landscape (as argued by studies such as Jenkins and Pimm (2003)), plant invaders can in turn alter their environment once established (Ehrenfeld 2003, Levine et al. 2003). Alterations to ecosystem functioning depends on how functionally distinct an invasive species is from the vegetation that it invades. Of course, there must be something that is allowing the invader to take over, and in many cases this is probably a superior ability to compete for a limiting resource, thus suggesting that nutrient cycling might become substantially altered when invasions occur. This holds whether the invaders are native or non-native. The ecosystem functions that are most often examined are carbon cycling, nitrogen cycling, hydrology, and fire regime (Ehrenfeld 2003, Levine et al. 2003). Changes to these ecosystem functions may affect the rate of invasion and the prospects of ecosystem return to a pre-invasion state. Some researchers have proposed that if a positive feedback between invader success and ecosystem alteration is formed, an "invasional meltdown" could occur where a new (possibly non-native) eco-order ascends to power (Simberloff and Von Holle 1999). Studies such as Lonsdale's (1999) suggest that this may not occur to its fullest extent: his most invaded ecosystem, Hawaii Volcanoes National Park, still had 36% native species, though it would be interesting to know relative non-native biomass and cover for that example as well as in other highly invaded systems.
Major environmental changes in grassland ecosystems like the introduction of livestock grazing and fire suppression often cause invasion by native and non-native woody plants, creating shrublands or woodlands (Archer 1994, Roques et al. 2001). Woody plants have been shown to alter the spatial distribution of nutrients (Schlesinger et al. 1990, Schlesinger and Pilmanis 1998, McCarron et al. 2003) and alter plant-soil water relations (Le Maitre et al. 2000). Nitrogen fixing invaders like Acacia saligna and Robinia pseudoacacia have been shown to drastically alter soil nitrogen status as they invade (e.g., Peloquin and Hiebert 1999). Fire-promoting grasses like Bromus tectorum and various non-native grasses in Hawaii increase fire frequency, thus promoting their own invasion (D'Antonio and Vitousek 1992, Knapp 1996). Tamarix ramosissima dominates riparian zones and transpires tremendous amounts of water (Graf 1978, Zavaleta 2000, Dahm et al. 2002).
Generalizing from specific examples such as these, Ehrenfeld (2003) reviewed the effects that invading plants may have on soil nutrient cycling processes, specifically the pools and fluxes of carbon and nitrogen that have been measured in association with non-native invasions. Plant traits that may be particularly important to pay attention to include size, canopy architecture, leaf area index, root structure, phenology, photosynthetic pathway, nutrient and water use efficiency, mycorrhizal associations, and secondary chemistry. Ehrenfeld found that most studies that looked for changes in carbon and nitrogen pools and flux rates between non-native invaders and native communities were able to demonstrate them, but there were no conspicuous trends when ecosystems became invaded: there were increases, decreases, and examples of very little change in the C and N pool and flux rates. Instead of offering final conclusions on the matter, Ehrenfeld proposes several "hypotheses to be tested" including that non-native species tend to cycle N more quickly and fix carbon more quickly. This goes well with other studies suggesting that non-native plants that are also invasive tend to demonstrate the traits associated with a ruderal strategy.
Most of the studies cited by Ehrenfeld show measurable changes, but it is difficult to determine just how substantial these changes are to overall ecosystem function, especially at larger scales. We need larger-scale, integrated measurements that will determine the effects of invasions on landscapes in order to determine if these types of measurable modifications will in fact create a positive feedback that will further alter physiognomy beyond the initial invasion.
Conclusion
Non-native plants, especially those that have become invasive, now make up a substantial fraction of Earth's vegetation. Important questions remain: Just how much surface cover, and just how much biomass consists of non-native plants? How much of what we see is non-native? Perhaps an operational definition of an invaded ecosystem would be useful. I would propose something like: Is a certain percentage of plant cover non-native? The sampling scale is of course an issue, but given the appropriate establishment of sampling scale, we would see that the alpine is not invaded and the riparian corridors of the west are highly invaded. Maybe 10% or maybe 25% of plant cover would be an appropriate cutoff. If we had enough data, we might see a satisfying breaking point that made ecological sense. What will happen to non-native cover in the future? It seems likely that it will increase as the environment of the Earth becomes further modified. Will it level off at a certain point where most dispersal events that will result in successful establishment will have occurred, or will the pace of modification stay high enough that non-natives start invading and replacing other non-natives? Will floras continue to be supplemented as has been occuring (more successful introductions than native extinctions), or will natives eventually start to drop out? How long might it take to reach what Stephen J. Gould called "the botanical equivalent of McDonald's" (Gould 1997)? Short of waiting around to see, some of these questions may be intractable, but their answers will have profound implications for the way our planet looks.
Is a physiognomy of invasive non-natives so different than one of pure natives? I grew up in Mountain View, California, where the mountains to be viewed are covered with invasive non-native annual grasses like Bromus diandrus. Had I not chosen to study biology, I might never have realized that coast live oaks (Quercus agrifolia) grew two centuries ago in a summer sea of green instead of brown. Still though, the brownness of the hills did not damp my appreciation for their beauty. Two centuries in the future, it will be intriguing to see if the hills have again taken on a new face.
Literature Cited
Archer, S. 1994. Woody plant encroachment into southwestern grasslands and savannas: Rates patters, and proximate causes. Pages 13-68 in M. Vavra, W. A. Laycock, and R. D. Pieper, editors. Ecological implications of livestock herbivory in the West. Society for Range Management, Denver, CO.
Callaway, R. M., and W. M. Ridenour. 2004. Novel weapons: invasive success and the evolution of increased competitive ability. Frontiers in Ecology and the Environment 2:436-443.
Cohen, J. E. 1995. How many people can the earth support?, 1st edition. Norton, New York.
Cronon, W. 1992. A Place for Stories: Nature, History, and Narrative. Journal of American History 78:1347-1376.
Cronon, W. 1995. The trouble with wilderness; or, getting back to the wrong nature. Pages 69-90 in W. Cronon, editor. Uncommon ground: toward reinventing nature. W.W. Norton & Co., New York.
Crosby, A. W. 1986. Ecological imperialism : the biological expansion of Europe, 900-1900. Cambridge University Press, Cambridge [Cambridgeshire] ; New York.
D'Antonio, C. M. 2000. Fire, plant invasions, and global changes. Pages 55-64 in H. A. Mooney and R. J. Hobbs, editors. Invasive species in a changing world. Island Press, Washington, D.C.
D'Antonio, C. M., and P. M. Vitousek. 1992. Biological invasions by exotic grasses, the grass/fire cycle, and global change. Annual Review of Ecology & Systematics 23:63-87.
Dahm, C. N., J. R. Cleverly, J. E. Allred Coonrod, J. R. Thibault, D. E. McDonnell, and D. J. Gilroy. 2002. Evapotranspiration at the land/water interface in a semi-arid drainage basin. Freshwater Biology 47:831-843.
Dukes, J. S., and H. A. Mooney. 1999. Does global change increase the success of biological invaders? Trends in Ecology & Evolution 14:135-139.
Ehrenfeld, J. G. 2003. Effects of exotic plant invasions on soil nutrient cycling processes. Ecosystems 6:503-523.
Ehrlich, P. R. 1968. The population bomb. Ballantine Books, New York,.
FAO. 2001. FAOSTAT land use database (http://apps.fao.org). Food and Agriculture Organization of the United Nations, Rome, Italy.
Galloway, J. N., W. H. Schlesinger, H. Levy, A. Michaels, and J. L. Schnoor. 1995. Nitrogen-Fixation - Anthropogenic Enhancement-Environmental Response. Global Biogeochemical Cycles 9:235-252.
Gould, S. J. 1997. An evolutionary perspective on strengths, fallacies, and confusions in the concept of native plants. Pages 11-19 in J. Wolschke-Bulmahn, editor. Nature and Ideology: Natural garden design in the twentieth century. Dumbarton Oaks Research Library and Collection, Washington, D.C.
Graf, W. F. 1978. Fluvial adjustment to the spread of tamarisk in the Colorado Plateau region. Geological Society of America Bulletin 89:1491-1501.
Hobbie, S. E. 1992. Effects of Plant Species on Nutrient Cycling. Trends in Ecology & Evolution 7:336-339.
Holm, L. G. 1997. World weeds : natural histories and distribution. Wiley, New York.
Houghton, J. T. 2001. Climate change 2001: the scientific basis: contribution of Working Group I to the third assessment report of the Intergovernmental Panel on Climate Change. Cambridge University Press, Cambridge ; New York.
Jenkins, C. N., and S. L. Pimm. 2003. How big is the global weed patch? Annals of the Missouri Botanical Garden 90:172-178.
Knapp, P. A. 1996. (Bromus tectorum L) dominance in the Great Basin Desert. History, persistence, and influences to human activities. Global Environmental Change Part A 6:37-52.
Lambers, H., F. S. Chapin, and T. L. Pons. 1998. Plant physiological ecology. Springer, New York.
Le Maitre, D. C., D. B. Versfeld, and R. A. Chapman. 2000. The impact of invading alien plants on surface water resources in South Africa: A preliminary assessment. Water SA 26:397-408.
Levine, J. M., M. Vila, C. M. D'Antonio, J. S. Dukes, K. Grigulis, and S. Lavorel. 2003. Mechanisms underlying the impacts of exotic plant invasions. Proceedings of the Royal Society of London - Series B: Biological Sciences 270:775-781.
Lonsdale, W. M. 1999. Global patterns of plant invasions and the concept of invasibility. Ecology 80:1522-1536.
Mack, R. N. 2000. Assessing the extent, status, and dynamism of plant Invasions: current and emerging approaches. Pages 141-168 in H. A. Mooney and R. J. Hobbs, editors. Invasive species in a changing world. Island Press, Washington, D.C.
Mack, R. N., and W. M. Lonsdale. 2001. Humans as global plant dispersers: Getting more than we bargained for. Bioscience 51:95-102.
Mack, R. N., D. Simberloff, W. M. Lonsdale, H. Evans, M. Clout, and F. A. Bazzaz. 2000. Biotic invasions: Causes, epidemiology, global consequences, and control. Ecological Applications 10:689-710.
McCarron, J. K., A. K. Knapp, and J. M. Blair. 2003. Soil C and N responses to woody plant expansion in a mesic grassland. Plant & Soil 257:183-192.
NRC. 2002. Abrupt climate change: inevitable surprises. National Research Council (U.S.). Committee on Abrupt Climate Change, Washington, D.C.
O'Neill, R. V. 2001. Is it time to bury the ecosystem concept? (With full military honors of course!). Ecology 82:3275-3284.
Odion, D. C., T. L. Dudley, and C. M. D'Antonio. 1988. Cattle Grazing in southeastern Sierran montane meadows: Ecosystem change and prospects for recovery. Pages 277-292 in C. A. Hall, V. Doyle-Jones, and M. DeDecker, editors. Plant biology of Eastern California: the Mary DeDecker Symposium. University of California White Mountain Research Station, Los Angeles, Calif.
Peloquin, R. L., and R. D. Hiebert. 1999. The effects of black locust (Robinia pseudoacacia L.) on species diversity and composition of black oak savanna/woodland communities. Natural Areas Journal 19:121-131.
Pimentel, D., L. Lach, R. Zuniga, and D. Morrison. 2000. Environmental and economic costs of nonindigenous species in the United States. Bioscience 50:53-65.
Pysek, P. 1995. On the terminology used in plant invasion studies. in Pysek, P.; Prach, K.; Rejmanek, M.; Wade, M.: Eds. Plant invasions: General aspects and special problems. . 1995. 71-81. SPB Academic Publishing bv, PO Box 97747, 2509 GC Amsterdam, Netherlands.
Roques, K. G., T. G. O'Connor, and A. R. Watkinson. 2001. Dynamics of shrub encroachment in an African savanna: Relative influences of fire, herbivory, rainfall and density dependence. Journal of Applied Ecology 38:268-280.
Scherer-Lorenzen, M., A. Elend, S. Nollert, and E.-D. Schulze. 2000. Plant invasions in Germany: general aspects and impact of nitrogen deposition. Pages 351-368 in H. A. Mooney and R. J. Hobbs, editors. Invasive species in a changing world. Island Press, Washington, D.C.
Schlesinger, W. H. 1997. Biogeochemistry: an analysis of global change, 2nd edition. Academic Press, San Diego, Calif.
Schlesinger, W. H., and A. M. Pilmanis. 1998. Plant-soil interactions in deserts. Biogeochemistry (Dordrecht) 42:169-187.
Schlesinger, W. H., J. F. Reynolds, G. L. Cunningham, L. F. Huenneke, W. M. Jarrell, R. A. Virginia, and W. G. Whitford. 1990. Biological Feedbacks in Global Desertification. Science 247:1043-1048.
Simberloff, D., and B. Von Holle. 1999. Positive Interactions of Nonindigenous Species: Invasional Meltdown? Biological Invasions 1:21-32.
Smith, S. D., T. E. Huxman, S. F. Zitzer, T. N. Charlet, D. C. Housman, J. S. Coleman, L. K. Fenstermaker, J. R. Seemann, and R. S. Nowak. 2000. Elevated CO2 increases productivity and invasive species success in an arid ecosystem. Nature 408:79-82.
Steltzer, H., and W. D. Bowman. 1998. Differential influence of plant species on soil nitrogen transformations within moist meadow Alpine tundra. Ecosystems 1:464-474.
Stevens, C. J., N. B. Dise, J. O. Mountford, and D. J. Gowing. 2004. Impact of nitrogen deposition on the species richness of grasslands. Science 303:1876-1879.
Vitousek, P. M., J. D. Aber, R. W. Howarth, G. E. Likens, P. A. Matson, D. W. Schindler, W. H. Schlesinger, and D. G. Tilman. 1997. Human alteration of the global nitrogen cycle: Sources and consequences. Ecological Applications 7:737-750.
Vitousek, P. M., C. M. DAntonio, L. L. Loope, and R. Westbrooks. 1996. Biological invasions as global environmental change. American Scientist 84:468-478.
Vitousek, P. M., and R. W. Howarth. 1991. Nitrogen Limitation on Land and in the Sea - How Can It Occur. Biogeochemistry 13:87-115.
Zavaleta, E. 2000. Valuing ecosystem services lost to Tamarix invasion in the United States. Pages 261-300 in H. A. Mooney and R. J. Hobbs, editors. Invasive species in a changing world. Island Press, Washington, D.C.
Zavaleta, E. S., M. R. Shaw, N. R. Chiariello, H. A. Mooney, and C. B. Field. 2003. Additive effects of simulated climate changes, elevated CO2, and nitrogen deposition on grassland diversity. Proceedings of the National Academy of Sciences of the United States of America 100:7650-7654.